1980's Marathi Medium Books Std 5 To 9
Sir aaple ekhade mobile app aahe ka, nasel tr please banva. Aaani tya through he avialable zhale tr. Ek navin kranti mpsc study madhe hoil. Thank you very much. All India Radio launched news-on-phone service on 25 February 1998 in New Delhi; it now has service in Chennai, Mumbai, Hyderabad, Indore, Patna and Bangalore. The service is accessible through Subscriber trunk dialing (STD), International Direct Dialing (ISD) and local calls. There are plans to establish the service in 11 additional cities.
AIR headquarters inAll India Radio ( AIR), officially known since 1956 as Ākāsha Vānī ('Voice from the Sky'), is the national radio broadcaster of and is a division of. It was established in 1930. It is the sister service of Prasar Bharati's, an Indian television broadcaster. Headquartered in the Akashvani Bhavan building in, it houses the Drama Section, the FM Section, the National Service, and is also home to the Indian television station Kendra,.All India Radio is the largest radio network in the world, and one of the largest broadcasting organizations in the world in terms of the number of languages broadcast and the spectrum of socio-economic and cultural diversity it serves. AIR’s home service comprises 420 stations located across the country, reaching nearly 92% of the country’s area and 99.19% of the total population. AIR originates programming in 23 languages and 179 dialects. Main article:(आकाशवाणी) is a word meaning 'celestial announcement' or 'voice from the sky/heaven'.
In, and, Akashvanis are often featured in stories as a medium of communication from heaven to mankind.' Akashvani' was first used in the context of radio by M. Gopalaswami in 1936 after setting up India's first private radio station in his residence, 'Vittal Vihar' (about two hundred yards from AIR’s current radio station). Akashvani was later adopted as All India Radio's on-air name in 1957.History Broadcasting began in June 1923 during the with programs by the and other radio clubs. According to an agreement on 23 July 1927, the private Indian Broadcasting Company Ltd (IBC) was authorized to operate two radio stations: the station which began on 23 July 1927, and the station which followed on 26 August 1927. The company went into liquidation on 1 March 1930.
The government took over the broadcasting facilities and began the Indian State Broadcasting Service (ISBS) on 1 April 1930 on an experimental basis for two years, and permanently in May 1932 it then went on to become All India Radio on 8 June 1936.On 1 October 1939, the External Service began with a broadcast in. It was intended to counter radio from Germany directed at Afghanistan, Persia and Arab nations. 1939 also saw the opening of the station of Eastern India, in what is now. This station catered and nurtured the pioneers of Bengali intellectuals. The foremost among them, became the trail-blazer of the talk-show in 1939.
He wrote and directed the first modern radio-play for this station in 1942. When India became independent in 1947, the AIR network had only six stations (, and ). The three radio stations at, and remained in what became Pakistan after the division. The total number of radio sets in India at that time was about 275,000. On 3 October 1957, the Service was launched, to compete with. Television broadcasting began in Delhi in 1959 as part of AIR, but was split off from the radio network as on 1 April 1976. FM broadcasting began on 23 July 1977 in, and expanded during the 1990s., the first radio station in (now ), went live on air on 3 February 1935.
It was launched by the 7th of Hyderabad with a transmitting power of 200. On 1 April 1950, Deccan Radio was taken over by the Indian Government, and in 1956 it was merged with All India Radio (AIR). Since then, it has been known as AIR-Hyderabad (100 kW). Domestic services AIR has many services in a number of languages, each serving different regions across India.Vividh Bharati is one of the best-known services of All India Radio. Its name roughly translates as 'Diverse Indian'.
It is also known as the Commercial Broadcasting Service or CBS. Commercially, it is the most accessible AIR network and is popular in and other large cities. Vividh Bharati offers a wide range of programs including news, film music, short plays, music and comedy. It operates on different -band frequencies in each city.Some programs broadcast on Vividh Bharati are:.: based on novels and plays. Santogen ki mehfil: ComedyOther services.
Primary Channel. National ChannelRegional services The headquarters of the Regional Deputy Directors General are located in Delhi and Chandigarh (NR), Lucknow and Bhopal (CR), Guwahati (NER), Kolkata (ER), Mumbai and Ahmedabad (WR), Chennai and Bangalore (SR). All India Radio.
Retrieved 13 January 2013. Retrieved 26 June 2018. (PDF). Archived from (PDF) on 17 September 2010. All India Radio.
Archived from on 12 June 2010. 15 August 2010.
Retrieved 30 July 2018. Archived from on 15 October 2011. Retrieved 2 May 2014. Archived from on 3 September 2011.
Retrieved 15 October 2011. IANS (31 August 2016).
1980's Marathi Medium Books Std 5 To 9 12
– via Business Standard. Retrieved 21 July 2016. Retrieved 17 October 2011.External links Wikimedia Commons has media related to.Look up in Wiktionary, the free dictionary.
NOTICE: This is an OSHA Archive Document, and may no longer represent OSHA Policy. It is presented here as historical content, for research and review purposes only.OSHA Instruction PUB 8-1.7 August 5, 1991 Directorate of Technical SupportSubject: Guidelines for Laser Safety and Hazard AssessmentA. This instruction provides guidelines to Federal OSHA and PlanStates compliance officers, 7(c)(1) consultants, and employee for theassessment of laser safety.B. This instruction applies OSHA-wide.C. Regional Administrators and Area Directors shall providecopies of the attached Guides for Laser Safety and Hazard Assessment to theappropriate State and Federal personnel and shall ensure that copies areavailable for distribution to the public upon request.D.
FEDERAL PROGRAM CHANGE.This instruction describes a change in the Federal program for whicha state response is not required. Each Regional Administrator, however,shall: 1. Ensure that this change is promptly forwarded to each statedesignee. Explain the technical content of this change to the state asrequested.
Inform the state designee that they are encouraged to makeavailable the Guidelines to State Plan personnel and appropriateemployers.E. STATE CONSULTATION PROJECTS.1. Regional Administrators shall forward a copy of thisinstruction to each consultation project manager and explain the technicalcontent when requested. Consultation Project Managers shall ensure that the informationin the Guidelines is provided to appropriate employers and ensure that copiesare available for distribution to the public upon request.F. With the increase development, manufacturing, and use ofdevices and systems based on stimulated emissions of radiation (Lasers) inindustrial applications, the compliance officer is now, more than ever, inneed of a comprehensive laser reference.
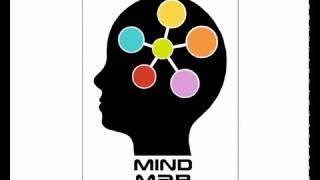
Because some primary users oftenmisunderstood the different orders of magnitude of intensity levels found inthe operational environment and the probability of potential accidentalexposure, it was necessary for this reference laser document to becomprehensive, easily read and understood.Gerard F. Scannell Assistant SecretaryDistribution:National, Regional and Area Offices State PlanDesignees 7(c)(1) Consultation Project Managers NIOSH Regional ProgramDirectors All Compliance OfficersGUIDELINESFOR LASER SAFETY ANDHAZARD ASSESSMENTOccupational Safety and Health Administration United States Department ofLabor Washington, DC 20210Table of ContentsI. Purpose of Guideline. I-1 B.Use of Guide. Who UsesLasers?. Laser Applications:. Projections for the 1990's:.
LASER TYPES AND OPERATION.II-1A. Basic Laser Operation.II-1 B.Energy Levels.II-1 C. RadiativeTransitions.II-1 D.
Absorption:.II-1 E. Spontaneous Emission:.II-1 F. Stimulated Emission.II-2 G.Population Inversion:.II-2 H. LaserComponents:.II-2 I. Specific Laser Types.II-5 J. Laser Beam Parameters.II-9 K.
Single frequency operation (monochromaticity).II-9 L. Point Source Emission. II-10 M.Gaussian Distribution of the Beam. Intensity of LaserEmission. Focused Laser Beams. Scanning Lasers.II-14 R.
II-15 S.Polarization of the Laser Output:. Electrical FieldStrength. Comparison With Other Sources. LASER HAZARDS. General Overview:.
III-1 B.Ultraviolet Effects on the Skin. Ocular Effectsof Laser Radiation. Extended Source Viewing. Eye Effects at Different Wavelengths:. Biological Damage Mechanisms:. III-7 G.Exposure Limits. MaximumPermissible Exposure Limits.III-11 I.
Associated (non-beam)Laser Hazards.III-11IV. LASER STANDARDS:.IV-1A. Overview of Standards.IV-1 B.Background of Laser Standards:. Standards VersusReality:.IV-4 D. Federal Laser ProductPerformance Standard (FLPPS).IV-5E. Reporting Guidelines (FLPPS):.IV-6V.
LASER CLASSIFICAITONS:. V-1 B.Laser Hazard Classes:. Optical FiberCommunication Systems (OFCS):. Laser ClassificationMeasurements. LASER HAZARD EVALUATION:.VI-1A.
Laser Environmental Factors.VI-1 B.Laser Safety Officer (LSO):.VI-1 C. StandardOperating Procedure:.VI-1 D. Laser Personnel.VI-1 E. The Nominal Hazard Zone.VI-2 F. Intrabeam Optical Density Determination.VI-8 G.Surgical Fiber OD Hazard Analysis.VI-8VII. CONTROL MEASURES. Control Measures-Overview.
VII-1 B.Laser Safety Officer:. Beam PathControls:. Laser Controlled Area.
Class IV Laser Controls-GeneralRequirements:. Entryway Control Measures (Class IV):. Administrative and Procedural Controls:. VII-6 H.Engineering Controls:. SafetyProcedures-General Basic Precautions.
Engineering ControlMeasures:.VII-11 K. Optical Fiber (Lightwave)Communication Systems (OFCS):.VII-13VIII.
PERSONAL PROTECTIVE EQUIPMENT.VIII-1A. Protective Equipment - Overview.VIII-1 B.Laser Protective Eyewear:.VIII-1 C. Selecting LaserEyewear.VIII-3 D. Selection Criteria:.VIII-3 E. Laser Output Factors:.VIII-4 F. Optical Density Determination.VIII-6 G.
EyeProtection for Support Staff and Spectators.VIII-7 H. Flashback Filtersfor Viewing Systems.VIII-7 I. Selection Process.VIII-7 J.
LASER TRAINING.IX-1A. Class I Training:.IX-1 B.Class II, Class IIA and Class IIIA Training.IX-1 C.
Class IIIB andClass IV Training:.IX-1 D. Laser Safety Officer Training.IX-2 E. Update Training Requirements:.IX-2 F. Tailored Training Sessions:.IX-2Appendix A. Glossary of Laser Terms:.
A-1Appendix B. Subject Index:. B-1Appendix C. C-1GUIDELINESFOR LASER SAFETY ANDHAZARD ASSESSMENTI. PURPOSE OF GUIDELINE:This guideline is designed to provide a general overview to lasers, laseruses, laser hazards and hazards analysis that are required to provideappropriate background for understanding the applicable industry standardsand regulatory requirements.B. USE OF GUIDE:The guide is divided into eleven topical sections.
Sections I to III providebackground information on laser and laser beams, laser bioeffects andancillary hazards. Pertinent definitions are in the glossary in Appendix A.Sections IV to VI cover the aspects of laser standards, classifications andoverall hazard analysis. Sections VII and VIII review laser controls andprotective equipment. Section IX covers training requirement.
A comprehensivereference listing is provided in Section X.In general, each section is designed to cover one major aspect of the laserstory and can be reviewed separately from the balance of the review. Thereis, of course, some need to have understanding of the earlier sections tofully apply information in the later sections, especially Section VII onlaser hazard evaluation.C. WHO USES LASERS?Estimates of the number of workers involved on a routine basis with laserdevises are difficult to perform. One method to estimate the number ofworkers is through the number of subscribers to the various laser relatedtrade magazines. Estimates indicate that the number of non-overlappingsubscribers to the three major laser/electro-optics magazines isapproximately sixty thousand. This number is based upon a comparativeevaluation of the total number of subscribers for each magazine using samplestatistical information for the number of non-overlapping subscribers.It should be stressed that these are controlled circulation magazines andare received by only 10-30% of the individuals at each facility involved withlaser and electro-optics activities.
Hence, one can estimate, using amultiplier ranging from three to ten, that the 'total' laser worker universein the early 1980's ranges from 180,000 to 600,000 people. Using estimatesof the projected growth over the next decade of 20% to 25% per year, one canproject a total laser-worker universe total in the early 1990's ranging from520,000 to perhaps as high as 6,000,000 people.A NIOSH report estimated that by 1980 about 9 million workers would havebeen potentially exposed to lasers and different arcs. These results werebased upon U.S.
Census Bureau estimates and other Government reports. Thisdata was divided into the following major census bureau occupationalcategories as shown in Table I-1.(Table I-1. Comparison of Occupational Category And Number of Workers, seeprinted copy)If it is assumed that only 60% of the workers are potentially exposed toarcs alone, this would mean that 3.6 million workers are potentially exposedto lasers alone.
This estimate is of the same basic magnitude as theestimate obtained previously (0.5 to 6 million) based upon magazinesubscription data. Comparison of these two estimates permits the generalconclusion that one can, with some certainty, conservatively project inexcess of one million workers involved with the applications of lasers by themid-to-late 1990's.Inspection of Table I-1 also indicates that the greatest percentage of thoseinvolved with lasers fall in the categories of craftsman, operator andservice. These represent approximately 89% of the total number.
This wouldindicate that the potential for accidental exposures to laser radiation willshift from the developmental engineer and scientist group (where a highpercentage of the previous incidents have occurred) to the generaloccupational work force. One might wonder how many more incidents willoccur with this shift to personnel who are much less aware of laser damages?D. LASER APPLICATIONS:The following will review some of the more important laser applications andtypes of lasers used. Emphasis will be placed upon those lasers with thelargest number of applications and, hence, involve the largest number ofworkers.
The major categories of laser uses are summarized in Table I-2.While the listing is extensive, it is certainly not exhaustive. The listingindicates numerous occupational and industry areas are involved in the laseruse. The heading of 'Research' was not included, although it is certainly amajor area for each of the categories listed as-well-as in the area of laserresearch itself.All of this indicates that potential for exposure to laser light hasexpanded beyond the scientific laboratory and workplace into theentertainment arena, museum, public building lighting, and even the home.E. PROJECTIONS FOR THE 1990'S:The current scope of laser applications is certainly extraordinary.Virtually every industry group is represented.
The question to be asked is,perhaps, 'WHAT NEW AREAS OF LASER APPLICATIONS WILL BE EXPLORED IN THE NEXTDECADE?' First, there will be the normal extension of the current applications acrossindustry lines.
Also, the use of higher power systems to serve multiple workstations on a beam time sharing basis will become more common. Most laserdevices will be dedicated systems, designed for a specific application.New applications will most probably center on the use of tunable wavelengthand ultraviolet laser devices (perhaps a second generation of excimerlasers). This lends itself to photochemistry and/or photobiological workwhere the need for a specific wavelength(s) is paramount for the application.Medical applications will be expanded with the use of various adjuvants withthe treatments. For example: dye injections will be administered to thepatient which are selectively absorbed in tumors to enhance the selectiveabsorption of laser energy in the tissues and provide a more specifictherapy.The uses of lasers with fiber optics will include, in addition tocommunications - which could become the singularly largest application areaof all laser uses - more uses in the industrial laser area. For example, thenatural extension of laser materials processing would be the incorporation oflaser fiber optics to conduct the beam to remote places as in the field ofrobotics.(Table I-2. Major Categories Of Laser Uses, see printed copy)All other new applications will bring certain unknowns from a laser hazardand overall occupational health point of view. For example, development ofthe Free Electron Laser (FEL), although now located at only a few isolatedresearch centers, has combined electron accelerator, high magnetic field andtunable laser technology together in a single installation.
In addition,research is underway for lasers to emit in the X-ray spectrum. All of thesedevelopments implies that the hazards associated with laser facilities willmost probably be more complex in the future.In addition, it is most probable that the use of the laser with newprocedures and processes will produce new and, perhaps, unknown substancesthat can present new hazards. It will be necessary, therefore that each ofthese new applications areas be approached with some caution and that thelaser interaction be studied and, where hazards are identified, methods ofcontrol be established.II. LASER TYPES AND OPERATIONA. BASIC LASER OPERATION:The term 'LASER' is an acronym.
It stands for 'Light Amplification byStimulated Emission of Radiation.' Thus the laser is a device which producesand amplifies light. The mechanism by which this is accomplished, stimulatedemission, was first postulated by Albert Einstein in 1917. The light whichthe laser produces is unique, for it is characterized by properties which arevery desirable, but almost impossible to obtain by any means other than thelaser.To gain a better understanding of the laser and what it can do, a review isincluded of some of the phenomena involved.B.
ENERGY LEVELS:Light can be produced by atomic processes, and it is these processes whichare responsible for the generation of laser light. Let's look first at atomicenergy levels and then see how changes in these energy levels can lead to theproduction of laser light.A number of simplifications will be made regarding the concept of the atom.It can be assumed, for the purposes of this discussion, that an atom consistsof a small dense nucleus and one or more electrons in motion about thenucleus.The relationship between the electrons and the nucleus is described in termsof energy levels. Quantum mechanics predicts that these energy levels arediscrete.C. RADIATIVE TRANSITIONS:The electrons normally occupy the lowest available energy levels. When thisis the case, the atom is said to be in its ground state.
However, electronscan occupy higher energy levels, leaving some of the lower energy statesvacant or sparsely populated.One way that electrons and atoms can change from one energy state to anotheris by the absorption or emission of light energy, via a process called aradiative transition.D. ABSORPTION:An electron can absorb energy from a variety of external sources. From thepoint of view of laser action, two methods of supplying energy to theelectrons are of prime importance. The first of these is the transfer of allthe energy of a photon directly to an orbital electron. The increase in theenergy of the electron causes it to 'jump' to a higher energy level; the atomis then said to be in an 'excited' state. It is important to note that anelectron can accept only the precise amount of energy that is needed to moveit from one allowable energy level to another. Only photons of the exactenergy acceptable to the electron can be absorbed.
Photons of slightly more(or slightly less) energy will not be absorbed.Another means often used to excite electrons is an electrical discharge. Inthis technique, the energy is supplied by collisions with electrons whichhave been accelerated by an electric field. The result of either type ofexcitation is that through the absorption of energy, an electron has beenplaced in a higher energy level than it originally resided. As a result, theatom of which it is a part is said to be excited.E. SPONTANEOUS EMISSION:The nature of all matter is such that atomic and molecular structures tendto exist in the lowest energy state possible. Thus, an excited electron in ahigher energy level will soon attempt to DE-EXCITE itself by any of severalmeans.
Some of the energy may be converted to heat.Another means of de-excitation is the spontaneous emission of a photon. Thephoton released by an atom as it is de-excited will have a total energyexactly equal to the difference in energy between the excited and lowerenergy levels. This release of a photon is called spontaneous emission. Oneexample of spontaneous emission is the common neon sign. Atoms of neon areexcited by an electrical discharge through the tube. They de-excitethemselves by spontaneously emitting photons of visible light.-NOTE: The exciting force is not of a unique energy, so that the electronsmay be excited to any one of several allowable levels.-Now let's look at the third, and probably the least familar, type ofradiative transition.F. STIMULATED EMISSION:In 1917, Einstein postulated that a photon released from an excited atomcould, upon interacting with a second, similarly excited atom, trigger thesecond atom into de-exciting itself with the release of another photon.
Thephoton released by the second atom would be identical in frequency, energy,direction, and phase with the triggering photon, and the triggering photonwould continue on its way, unchanged. Where there was one photon now thereare two.
These two photons could then proceed to trigger more through theprocess of stimulated emission.If an appropriate medium contains a great many excited atoms andde-excitation occurs only by spontaneous emission, the light output will berandom and approximately equal in all directions. The process of stimulatedemission, however, can cause an amplification of the number of photonstraveling in a particular direction - a photon cascade if you will.A preferential direction is established by placing mirrors at the ends of anoptical cavity. Thus the number of photons traveling along the axis of thetwo mirrors increases greatly and Light Amplification by the StimulatedEmission of Radiation may occur. If enough amplification occurs, LASER beamis created.G. POPULATION INVERSION:Practically speaking, the process of stimulated emission will not produce avery efficient or even noticeable amplification of light unless a conditioncalled 'population inversion' occurs. If only a few atoms of several millionare in an excited state, the chances of stimulated emission occurring aresmall.
The greater the percentage of atoms in an excited state, the greaterthe probability of stimulated emission. In the normal state of matter thepopulation of electrons will be such that most of the electrons reside in theground or lowest levels, leaving the upper levels somewhat depopulated.
Whenelectrons are excited and fill these upper levels to the extent that thereare more atoms excited than not excited, the population is said to beinverted.H. LASER COMPONENTS:A generalized laser consists of a lasing medium, a 'pumping' system and anoptical cavity.
The laser material must have a metastable state in which theatoms or molecules can be trapped after receiving energy from the pumpingsystem. Each of these laser components are discussed below:1. PUMPING SYSTEMS: a.
The pumping system imparts energy to the atoms or moleculesof the lasing medium enabling them to be raised to an excited 'metastablestate' creating a population inversion. Optical pumping uses photonsprovided by a source such as a Xenon gas flash lamp or another laser totransfer energy to the lasing material. The optical source must providephotons which correspond to the allowed transition levels of the lasingmaterial. Collision pumping relies on the transfer of energy to thelasing material by collision with the atoms (or molecules) of the lasingmaterial. Again, energies which correspond to the allowed transitions must beprovided. This is often done by electrical discharge in a pure gas or gasmixture in a tube. Chemical pumping systems use the binding energy released inchemical reactions to state.
OPTICAL CAVITY: An optical cavity is required to provide the amplificationdesired in the laser and to select the photons which are traveling in thedesired direction. As the first atom or molecule in the metastable state ofthe inverted population decays, it triggers via stimulated emission, thedecay of another atom or molecule in the metastable state.
If the photonsare traveling in a direction which leads to the walls of the lasing material,which is usually in the form of a rod or tube, they are lost and theamplification process terminates. They may actually be reflected at the wallof the rod or tube, but sooner or later they will be lost in the system andwill not contribute to the beam. If, on the other hand, one of the decaying atoms or moleculesreleases a photon parallel to the axis of the lasing material, it can triggerthe emission of another photon and both will be reflected by the mirror onthe end of the lasing rod or tube. The reflected photons then pass backthrough the material triggering further emissions along exactly the same pathwhich are reflected by the mirrors on the ends of the lasing material. Asthis amplification process continues, a portion of the radiation will alwaysescape through the partially reflecting mirror. When the amount ofamplification or gain through this process exceeds the losses in the cavity,laser oscillation is said to occur. In this way, a narrow concentrated beamof coherent light is formed.Table II-1 Most Common Laser Wavelengths (Table II-1.
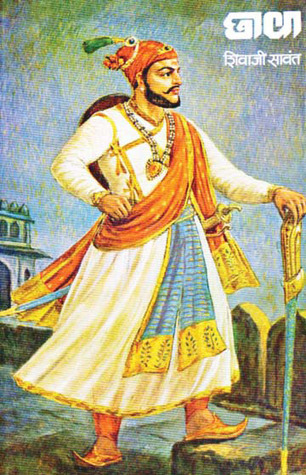
Most Common Laser Wavelengths, see printed copy) The mirrors on the laser optical cavity must be preciselyaligned for light beams parallel to the axis. The optical cavity itself,i.e., the lasing medium material must not be a strong absorber of the lightenergy. LASER MEDIA: Lasers are commonly designated by the type of lasing materialemployed. There are four types which are: solid state, gas, dye, andsemiconductor. The characteristics of each type will be described. Note thewavelengths in Table II-1. SOLID STATE LASERS employ a lasing material distributed in asolid matrix.
One example is the Neodymium: YAG laser (Nd:YAG). The term:YAG is an abbreviation for the crystal: Yttrium Aluminum Garnet which servesas the host for the Neodymium ions. This laser emits an infrared beam at thewavelength of 1.064?m (?m = 10(-6) meters). Accessory devices that may beinternal or external to the cavity may be used to convert the output tovisible or ultraviolet wavelength. GAS LASERS use a gas or a mixture of gases within a tube.The most common gas laser uses a mixture of helium and neon (HeNe), with aprimary output of 632.8 nm (nm = 10(-9) meter) which is a visible red color.It was first developed in 1961 and has proved to be the forerunner of a wholefamily of gas lasers. All gas lasers are quite similar in construction andbehavior. For example, the carbon dioxide (CO(2)) gas laser radiates at 10.6?m in the far-infrared spectrum.
Argon and krypton gas lasers operate withmultiple frequency emissions principally in the visible spectra. The mainemission wavelengths of an argon laser are 488 and 514 nm. DYE LASERS use a laser medium that is usually a complexorganic dye in liquid solution or suspension. The most striking feature ofthese lasers is their 'tunability.' Proper choice of the dye and its concentration allows theproduction of laser light over a broad range of wavelengths in or near thevisible spectrum. Dye lasers commonly employ optical pumping although some typeshave used chemical reaction pumping. The most commonly used dye is Rhodamine6G which provides tunability over 200 nm bandwidth in the red portion (620nm) of the spectrum.
SEMICONDUCTOR LASERS (sometimes referred to as diode lasers)are not to be confused with solid state lasers. Semiconductor devicesconsist of two layers of semiconductor material sandwiched together. Theselasers are generally very small physically, and individually of only modestpower. However, they may be built into larger arrays.
The most common diodelaser is the Gallium Arsenide diode laser with a central emission of 840nm. TIME MODES OF OPERATION: The different time modes of operation of a laser aredistinguished by the rate at which energy is delivered. CONTINUOUS WAVE (CW) lasers operate with a stable averagebeam power. In most higher power systems, one is able to adjust the power. Inlow power gas lasers, such as HeNe, the power level is fixed by design andperformance usually degrades with long term use. SINGLE PULSED (normal mode) lasers generally have pulsedurations of a few hundred microseconds to a few milliseconds. This mode ofoperation is sometimes referred to as long pulse or normalmode.
SINGLE PULSED Q-SWITCHED lasers are the result of anintracavity delay (Q-switch cell) which allows the laser media to store amaximum of potential energy. Then, under optimum gain conditions, emissionoccurs in single pulses; typically of 10(-8) second time domain.
These pulseswill have high peak powers often in the range from 10(6) to 10(9) Wattspeak. REPETITIVELY PULSED or scanning lasers generally involve theoperation of pulsed laser performance operating at a fixed (or variable)pulse rates which may range from a few pulses per second to as high as 20,000pulses per second. The direction of a CW laser can be scanned rapidly usingoptical scanning systems to produce the equivalent of a repetitively pulsedoutput at a given location. MODE LOCKED lasers operate as a result of the resonant modesof the optical cavity which can effect the characteristics of the outputbeam. When the phases of different frequency modes are synchronized, i.e.,'locked together,' the different modes will interfere with one another togenerate a beat effect. The result is a laser output which is observed asregularly spaced pulsations. Lasers operating in this mode-locked fashion,usually produce a train of regularly spaced pulses, each having a duration of10(-15) (femto) to 10(-12) (pico) sec.
- Smitemedia-Want to see more let's plays of ProjectSmite? Hd1duration16:38publishedJanuary 13, 2014descriptionHey there guys, and welcome to my new show called - civilization 5 mod showcase, where i'll be showcases random mods that I find on the steam workshop!. YOU CAN ALSO PLAY ON THE MAP 'TAMRIEL' THE OFFICIAL ELDER SCROLLS MAP BY PLAYING THROUGH THE SCENARIO SCREEN!So, on this episode we're looking at the elder scrolls universe civilizations, and their leaders. Civ 5 map mods. More info and mod download here -Civilizations.Cyrus (Hammerfell).Gortwog gro-Nagorm (Orsinium).Haymon Camoran (Valenwood).High King Emeric (High Rock).Hlaalu Helseth (Morrowind).Keirgo (Elsweyr).King Dumac (Dwemereth).Mehrunes Dagon (Oblivion).Queen Ayrenn (Summerset Isles).The An-Xileel (Argonia).Tiber Septim (Cyrodiil).Umaril the Unfeathered (The Ayleids).Ysgramor (Skyrim)Check out our website and join in the discussions!
A mode-locked laser can deliverextremely high peak powers than the same laser operating in the Q-switchedmode. These pulses will have enormous peak powers often in the range from10(12) Watts peak.I. SPECIFIC LASER TYPES:1. HELIUM NEON LASER: The first CW system was the helium neon (HeNe) gas mixture.Although its first successful operation was at an infrared wavelength of 1.15?m, the HeNe laser is most well known operating at the red 633 nm transition.Some HeNe lasers today also can emit operate at other wavelengths (594 nm,612 nm, 543 nm). Some earlier HeNe lasers were excited by radio frequency(RF) discharge but virtually all HeNe lasers today are driven by a small DCdischarge between electrodes in the laser tube. The HeNe laser operates by an excitation of the helium atomsfrom the ground state.
This energy excess is coupled to an unexcited neonatom by a collisional process with the net result of an inversion in the neonatom population, thus allowing laser action to begin. Power levels availablefrom the HeNe laser ranges from a fraction of a milliwatt to about 75milliwatts in the largest available systems. The HeNe laser is noted for itshigh-frequency stability and TEM(oo) (single mode) operation. The HeNe laseris one of the most widely used laser in existence today.
Its pencil-thinbeam is used in surveying work, to align pipelines, as a sawing guide insawmills, and is also used to 'align' patients in medical X-ray units, justto name a few of its many applications. It is also used in many retailscanners, lecture hall pointers and display devices. In addition, hologramsare often made using the coherent light of HeNe lasers. ARGON, KRYPTON AND XENON ION LASERS The family of ion lasers utilize argon, krypton, xenon, and neongases to provides a source for over 35 different laser frequencies, rangingfrom the near ultraviolet (neon at 0.322?m) to the near-infrared (krypton at0.799?m). It is possible to mix the gases, for example, argon and krypton,to produce either single frequency or simultaneous emission at ten differentwavelengths, ranging from the violet through the red end of thespectrum.
The basic design of an ion gas laser is similar to the HeNe. Themajor difference is that the electrical current flowing in the laser tubewill be 10-20 amperes; sufficient to ionize the gas. Population inversion isobtained only in the ionized state of the gas. An important feature of theselasers is the very stable (0.2%) high output power of up to 20 Watts/CW.Commercial models will normally have a wavelength selector (a prism) withinthe cavity to allow for operation at any one of the wavelengths available.In addition, approximately single frequency operation can be achieved byplacing an etalon inside the optical resonator cavity. Argon ion lasers produce the highest visible power levels andhave up to 10 lasing wavelengths in the blue-green portion of the spectrum.These lasers are normally rated by the power level (typically 1-10 Watts)produced by all of the six major visible wavelengths from 458 to 514 nm.
Themost prominent argon wavelengths are the 514 and 488 nm lines. Wavelengthsin the ultraviolet spectrum at 351 and 364 nm available by changing resonatormirrors. To dissipate the large amount of generated heat, the larger argonion laser tubes are water cooled.
Although some lasers have separate heatexchangers, most use tap water. Simple pulsed versions of argon ion lasers also are available.Since the duty cycle ('on' time divided by the time between pulses) is low,the heat energy generated is small, and usually only convective cooling isneeded. The average power output may be as high as several Watts, thoughtthe peak powers can be as high as several kilowatts. Pulse widths areapproximately five to fifty microseconds, with repetition rates as high as 60Hz. CARBON DIOXIDE LASER The carbon dioxide laser is the most efficient and powerful ofall CW laser devices. Continuous powers have been reported above 30kilowatts at the far infrared 10.6?m wavelength.
An electrical discharge is initiated in a plasma tube containingcarbon dioxide gas. CO(2) molecules are excited by electron collisions tohigher vibrational levels, from which they decay to the metastablevibrational level occurs; which has a lifetime of approximately 2x10(-3)seconds at low pressure of a few Torr. Establishing a population inversionbetween certain vibrational levels leads to lasing transitions at 10.6?m,while a population inversion between other vibrational levels can result inlasing transitions at 9.6?m.
Although lasing can be obtained in a plasmatube containing CO(2) gas alone, various gases usually added, including N(2),He, Xe, CO(2) and H(2)O. Such additives are used to increase the operatingefficiency of CO(2) lasers. The most common gas composition in CO(2) lasersis a mixture of He, N(2) and CO(2). Carbon dioxide lasers are capable of producing tremendousamounts of output power, primarily because of the high efficiency of about30%, as compared to less than 0.1% for most HeNe lasers.
The principaldifference between the CO(2) and other gas lasers is that the optics must becoated, or made of special materials, to be reflective or transmissive at thefar infrared wavelength of 10.6?m. The output mirror can be made ofgermanium, which, if cooled, has very low loss at 10.6?m. There are three common laser cavity configurations of the CO(2)laser. The first is the gas discharge tube encountered with the discussionof the HeNe laser. Secondly is the axial gas flow, where the gas mixture ispumped into one end of the tube and taken out the other. The gas flow allowsfor the replacement of the CO(2) molecules depleted (disassociated CO(2)molecules) by the electrical discharge.
Nitrogen is added to the CO(2) toincrease the efficiency of the pumping process and transfers energy bycollisions. Associated effects enhance the de-excitation process. Helium isadded to the mixture to further increase the efficiency of the process ofpumping and stimulated emissions. The third method is the transverse gasflow. This technique can produce CO(2) laser emissions at power levelsapproaching 25 kW. The CO(2) laser has a strong emission wavelength at 10.6 micrpm.
There is another strong line at 9.6 miceo m and a multitude of linesbetween 9 and 11?m. CO(2) lasers are highly efficient (10-30%), give highoutput powers (used for welding and cutting), and applications out-of-doorscan take advantage of low transmission loss atmospheric window at about 10?m. ND:YAG LASER SYSTEMS: One of the most widely used laser sources for moderate to highpower uses a neodymium doped crystal Yttrium Aluminum Garnet (YAG), commonlydesignated Nd:YAG.
In addition, other hosts can be used with Nd, such ascalcium tungstate and glass. The Nd:YAG laser is optically pumped either bytungsten or krypton pump lamps and is capable of CW outputs approaching 2000W at the 1.06?m wavelength. The ends of the crystal, which is usually in theform of a rod, are lapped, polished, and may be coated to provide the cavitymirrors. Nd:YAG lasers belong to the class of solid state lasers. Solidstate lasers occupy a unique place in laser development. The firstoperational laser medium was a crystal of pink ruby (a sapphire crystal dopedwith chromium); since that time, the term 'solid state laser' usually hasbeen used to describe a laser whose active medium is a crystal doped with animpurity ion.
Solid state lasers are rugged, simple to maintain, and capableof generating high powers. Although solid state lasers offer some unique advantages overgas lasers, crystals are not ideal cavities or perfect laser media. Realcrystals contain refractive index variations that distort the wavefront andmode structure of the laser. High power operation causes thermal expansionof the crystal that alters the effective cavity dimensions and thus changesthe modes. The laser crystals are cooled by forced air or liquids,particularly for high repetition rates. The most striking aspect of solid state lasers is that theoutput is usually not continuous, but consists of a large number of oftenseparated power bursts.
Normal mode and Q-Switched solid-state lasers areoften designed for a high repetition-rate operation. Usually the specificparameters of operation are dictated by the application. For example, pulsed YAG lasers operating 1 Hz at 150 Joules perpulse are used in metal removal applications. As the repetition rateincreases, the allowable exit energy per pulse necessarily decreases.Systems are in operation, for example, which produce up to ten Joules perpulse at a repetition rate of 10 Hz.
A similar laser, operated in theQ-Switched mode, could produce a one megawatt per pulse at a rate up to tenpulses a minute. EXCIMER LASERS: High power ultraviolet (UV) lasers have been the desire of manyin the laser applications community for over twenty-five years.Theoretically, such a laser could produce a focused beam of sub-micrometersize and, therefore, be useful in laser microsurgery and industrialmicrolithography. Also, photochemical processes which are dependent upon theshorter UV wavelength would be possible at significantly greater speedsbecause of the enormous UV photon flux presented by a laserbeam. In 1975 the first of a family of new UV laser devices wasdiscovered by Searles and Hart.
This type laser was to be referred to as anexcimer laser, an abbreviation for the term: Excited Dimer. It has takenabout a decade for these devices to move from the development lab into realworld applications.
Excimer lasers operate using reactive gases such as chlorine andfluorine mixed with inert gases such as argon, krypton or xenon. The variousgas combinations, when electrically excited, produce a pseudo molecule(called a 'dimer') with an energy level configuration that causes thegeneration of a specific laser wavelength emission which falls in the UVspectrum as given in Table II-2.
The reliability of excimer lasers has madesignificant strides over the past several years. Now, systems operating ataverage powers from 50-100 Watts are commercially available. A typicalexcimer operates in a repetitively pulsed mode of 30-40 ns pulses at pulserates up to 50 Hz with pulse energies of 1-2 Joules/pulse. Some systems usex-rays to preionize the excimer laser's gas mixture so-as-to enhance lasingefficiency and increase the overall output power. EXCIMER LASE WAVELENGTHS (Table II-2.
Excimer Lase Wavelengths, see printed copy) Until the late 1980s, excimer lasers were more commonly found inthe research laboratory where they are used either as a specific UV sourceor, in many cases, to serve as a 'pumping' or exciting source to generatevisible laser emissions. In the latter case, the excimer's UV output isdirected into a tunable dye laser or Raman shifter module and converted intoa modestly high power visible frequency emission. LASER TYPES FOR CURRENT APPLICATIONS(Table II-3. Laser Types for Current Applications, see printed copy)Excimer lasers are now making the transition from the lab to theproduction area for a few unique uses in industry or in the operating roomfor exploratory surgical applications. SEMICONDUCTOR DIODE LASERS The semiconductor or diode injection laser is another type ofsolid state laser. The energy level scheme is constructed by charge carriersin the semiconductor.
They may be pumped optically or by electron beambombardment, but most commonly, they are pumped by an externally appliedcurrent. Although all of these devices operate in the near infrared spectralregion,visible laser diodes are being made today.
A useful feature is thatmany are tunable by varying the applied current, changing temperature, or byapplying an external magnetic field. Laser diodes are used extensively forcommunications, in compact disc players, retail scanners, printer, and arebeginning to be used in ophthalmology. A summary of applications of the more common lasers is given inTable II-3. Semiconductor lasers are used in distance detectors and remotesensing systems, rangefinders, and for voice and data communications. Manyof the diode lasers may be operated on a continuous wave basis. The mostcommon diode uses a gallium-arsenide junction which emits a fan shapedinfrared beam at 840 nm. OTHER LASERS: Dye Lasers were the first true tunable laser.
Using differentorganic dyes, a dye laser is capable of producing emission from theultraviolet to near infrared. Most are operated in the visible with tunableemissions of red, yellow, green, or blue laser emission at almost anywavelength. The more common organic dye lasers are optically pumped.
Themost common dye used is Rhodamine-6G in solution. Such lasers may either beflashlamp pumped, or more commonly pumped with another laser such as an Argonor Nitrogen laser. To obtain CW reliable operation the dye is made to flowthrough a thin cell. Using the appropriate dye solutions, an argon-ion laseras a pump, and a prism, the dye laser is tunable across most of the visiblespectrum. Tunable dye lasers are now widely used in high resolution atomicand molecular spectroscopy.J. LASER BEAM PARAMETERS:The following seven properties are common to the beams emitted from alllaser types and are the factors which, when combined together, distinguishlaser outputs from other sources of electromagnetic radiation:1. A nearly single frequency operation of low bandwidth (i.e., analmost pure monochromatic light beam).
A beam with a Gaussian beam intensity profile. A beam ofsmall divergence. A beam of enormous intensity. A beam whichmaintains a high degree of temporal and spatial coherence.
A beam that is, in many laser devices, highly planepolarized. A beam with enormous electromagnetic field strengths.Each of these laser beam properties are briefly reviewed in the followingsections.K. SINGLE FREQUENCY OPERATION (MONOCHROMATICITY):The frequency of any electro-magnetic wave is related to the number ofcycles the electric or magnetic field undergo each second. A completelycoherent, monochromatic wave oscillates exactly at a constant frequency.Most laser systems display a narrow multifrequency characteristic. Thisfrequency spread is, however, very narrow when compared to the average laserfrequency.In most lasers, the frequency degeneracy is solely dependent upon thequantum transition characteristics of the active media, and the geometry ofthe laser resonator cavity (Fabry-Perot). In this sense, the laser media maybe considered as a high number of isolated light generators placed betweentwo mirrors. The electromagnetic field developed between the mirrors may beregarded as a superposition of plane waves at each of the slightly differentfrequencies which the laser media generates and allows to oscillate.
Thesedifferent frequencies are termed the 'modes' of the laser resonator. Theoff-axis modes result from plane waves propagating at an angle with respectto the axis of the resonator. These different modes are produced bydiffraction effects in the Fabry-Perot cavity.
The lowest order axial modeis designated as the TEM(oo) mode. This mode has the lowest diffractionlosses and often will be the predominant mode of oscillation.For each transverse mode, there will be many longitudinal modes which canoscillate; hence the output of a multimode laser will actually contain asuperposition of plane waves oscillating at many discrete frequencies.However, as previously mentioned, this frequency spread will be very small.In each laser, there will be specific 'allowed' frequencies of the resonatorcavity (Fabry-Perot modes). For most cases, the average wavelength at whichthe laser oscillates is sufficient to describe it's operation. If moreprecision is needed, then the frequency spread or bandwidth is given.Depending on the type of laser, bandwidths range typically from 10(-4) to10(-9) times the average frequency of the laser; although bandwidths as lowas 0.1 Hz. Have been reported for stabilized gas lasers.The wave nature of light most often allows adequate description of theoutput of the laser, and for most cases it will be sufficient to usegeometrical optics to describe the output as a beam with well defined edgesand some beam divergence. The beam is emitted from the laser with a beamdiameter (alpha) and a beam divergence (PHI), as though it came from a smallpoint source far behind the laser output aperture.L. POINT SOURCE EMISSION:The emission from most lasers can be considered as emanating from a 'virtualpoint source' located within or behind the laser device.
A 'virtual pointsource' is one which really doesn't exist, but the properties of the emittedbeam are such that there appears to be a source at this position.The distance of the point source behind the front mirror of the laser can berelated to the size of the beam at the mirror (X) and the laser beamdivergence (phi) by the equation:(Equation, see printed copy)Thus, the virtual point source is located two meters behind the exit mirror.M. GAUSSIAN DISTRIBUTION OF THE BEAM:The intensity profile across a TEM(oo) laser beam will be in the form of abell-shaped (Gaussian) distribution. The decrease in intensity at the edge ofthe beam is the result of diffraction effects produced at the edges.The spatial intensity distribution of this mode may be expressed by equation:(Equation, see printed copy)where R is the radius and W is a constant which defines the mean radius andis commonly referred to as the 'spot size.'
At this point the intensity hasfallen to e(-2) of the peak intensity at the center of distribution.In fact, the edges of the laser beam are not well defined. If one were tomeasure the energy or power per unit area point by point across the center ofthe output aperture, a Gaussian beam distribution is defined. The peakintensity is in the center of the beam and approaches zero as one moves fromthe center.
This shape is maintained as the beam propagates through spacesubject to broadening and distortion by atmospheric effects.Important points on the distribution curve are the e(-1) and e(-2) intensitypoints since they are used as standard quantities to define the laser beamdivergence parameter. (The e is the natural number associated with thenatural logarithm and is equal to: e= 2.7183). The e(-1) point is where theintensity is reduced by the factor(Equation see printed copy)or approximately 63% of the energy (or power) is contained within theaperture of diameter (a) centered in the beam.-Note: In most all laser safety and compliance standards, the outputaperture, a, and the beam divergence, (PHI), are defined relative to thee(-1) points. The total laser energy (Q(t)) or beam power (PHI) is definedas that which is collected from the entire beam (total values).-Many manufactures specifications use the e(-2) i points to define beamdivergence.
In this case, e(-2) i = 0.1353, or the total power (energy) is:100% - 0.13 3 x 100% = 86.47% or approximately 86% of the total energy /poweris within the e(-2) aperture. In some cases, they specify relative to the90% point.
Note that the beam divergence is larger at the e(-2) or 90%point. Hazard calculations are sensitive to the beam divergence andconversions from e(-2) points to e(-2) power points are often performed onbeam sizes.The beam diameters at the two points are related:(Equation, see printed copy)Departure from the Gaussian distribution arise when independent oscillationoccurs within the resonator at higher order modes. For example, some gaslasers may be designed to have sufficient gain to support simultaneousoscillation in many different transverse modes. Mode selection may often beaccomplished by slight adjustment of the mirror alignments.
With thistechnique, one can observe the different complex intensity distributions ofeach mode.The lowest order TEM(oo) mode with the nearly Gaussian intensitydistribution has the lowest cavity losses and hence will generally be thedominant mode of oscillation.Optically pumped solid-state lasers such as the normal mode Nd:YAG laserusually display a randomly varying mode output. Thermal gradients in theoptical media (i.e., the crystal) caused by nonuniform absorption of the pumplight give rise to lens effects in the crystal which change during thepumping cycle. The result is a sporadic switching of transverse modes duringthe laser pulse. The time average is generally a bell-shaped distributionwhich is dependent upon the optical purity of the laser crystal, the pumpingscheme, and the level at which the system is operated above lasing threshold.Some pumping schemes produce pronounced 'hot spots' in the intensitydistributions. For long range transmission, atmospheric effects can alsoproduce intensity variations by a factor of ten over localized regions of thebeam. Such non-uniformities distribution make it difficult to specify thecross sectional area of the beam. As a result, an average value of beamradius must be chosen.
Typically, this is often (1) the half-power point;(2) the e(-1) power point; or (3) the e(-2) power point.A more precise laboratory practice is to measure the diameter at the statedpower point on a densitometer recording obtained from a photographic negativeof the output beam distribution.In the case of optically pumped solid-state lasers, the size of the beamcross section is generally a function of the pumping level of the laser. Ingeneral, the higher the pumping level, the wider the beam size.
Only whenpulsed lasers are operated near threshold, or in special cavity conditions,will the zero order mode (lowest beam spread) predominate.N. BEAM DIVERGENCEBeam divergence is a very important laser parameter and is often expressedin units of milliradians. The symmetry of the laser beam allows the geometryto be reduced to the two dimensions of a plane. The angle (phi), in radianscan be related to degrees by noting that for a full circle, phi is 360degrees.For some smaller angle, the arc length(s) intercepted along thecircumference of the circle can be used to define the angle as:(Equation, see printed copy)The minimum beam divergence, called the diffraction limited beam divergence,is related by the equation:(Equation, see printed copy)This concept is expanded to three dimensions by introducing the concept ofsolid angle. The solid angle (OMEGA) is expressed in units of steradians (sr)and is determined by using the area cut out of a surface of a sphere dividedby the square of the distance to that surface; that is:(Equation, see printed copy)For a sphere, the solid angle may be opened up to include the entire spheresurface area (A = 4 pi R(2)), therefore:(Equation, see printed copy)The output of a typical laser will be confined to less than 10(-6) sr.O. INTENSITY OF LASER EMISSION:In many applications, the most important laser beam charactertic is theenormous intensity of the beam. Intensity is related to the beam power thecross sectional area and the manner in which the beam spreads from one pointin space to the next.Power, by definition, is the time-rate at which work is done; specifically,it is the rate at which energy is used or produced.
1980's Marathi Medium Books Std 5 To 9 1
Energy relates theability to do work. As with other forms of energy (eg, chemical, mechanical,electrical), electromagnetic energy (light energy) is a conserved quantity.The relationship between energy, power, and time is defined by the integralequation:(Equation see printed copy)The intensity of the laser is usually expressed by the IRRADIANCE(power/area) of the beam. This is determined by dividing the average valueof beam power by the average value of the beam cross section. Irradianceunits are expressed in Watts per square centimeter.In pulsed laser operation, instantaneous (peak) Irradiances in excess of100,000 W/cm(2) are quite easily generated in an unfocused high energy pulsedsolid state laser pulse. If this output were contained within a typical beamdivergence of 20 milliradians and focused by only moderate power optics, theIrradiance at the focal plane would be increased at least one-hundred fold.A CW laser is rated in Watts and a pulsed laser is normally rated accordingto the total energy (Joules) per pulse. Pulsed outputs are also expressed asa RADIANT EXPOSURE in units of Joules per square centimeter.In order to determine the peak power of pulsed laser, it is necessary toknow the pulse shape and duration.